One of the main problems with neonicotinoids is that they have a delayed toxic effect that renders small residual concentrations of the chemicals especially problematic for long-lived beneficial insects such as bees. The argument is simple enough once you grasp the implications of delayed toxicity. Allow me to digress and offer a comparison of toxic time dependence and example chemicals.
1) Threshold toxicity. Consider CO2 – usually not a poison unless there is so much of the gas that you cannot get enough oxygen to breathe. If the concentration of CO2 got high enough to deprive you of oxygen, it would kill you. However, even long exposure at levels 30% of the toxic threshold are likely to have little effect. Typical insecticides that work this way are dormant oil sprays that essentially suffocate the target insects by plugging up their tracheal breathing tubes.
2) Linear accumulation – Haber’s Rule. Some toxins exhibit “accumulation to a threshold” behavior. In the 1900′s Fritz Haber noticed this effect with the concentration of chlorine gas. The concentration of the gas multiplied by the length of exposure produced the same lethal effect. The lethal concentration varies as 1/t where t is the exposure time, or LC50 = k/t where LC50 is the concentration that kills half of the subjects and k is proportionality constant. Several insecticides have this behavior, including many of the old fashion organophosphate pesticides. This all makes a certain amount of sense, because if you add up the total exposure over time, you find it takes the same total amount of toxin to kill the organism, proportionally longer if you use proportionally less concentrated toxin.
3) Delayed and Enhanced Toxicity – We can write out a scaling law as LC50 = k/t˄p. When the time exponent p=0, then we have case 1) above where the toxic threshold is k. When p=1 we have Haber’s Rule, case 2) above. When p>1 we have a situation where the toxic concentration required to kill half of the target organisms is less if the exposure is longer, and furthermore, the total accumulated toxin required to kill the organism is less the longer you wait. Such enhanced toxicity can come about because several conditions about how the toxin operates are met. These included 1) strong binding for the toxic molecules to the sites of activity in the organism, 2) direct action of the toxin biologically (no threshold required to damage biological function) and 3) biological accumulated effects of the toxin. The neonicotinoids often fit exactly these criteria. It is not a standard requirement to evaluate the toxicity time-scaling of pesticides, although it should be. I looked carefully at the literature and found several studies that, taken together, point to approximately a 1/t2 scaling dependence for imidacloprid with bees.
For honeybees, the LD50 for imidacloprid is about 40ng/bee, or nectar and pollen concentrations around 800 parts per billion (ppb). If we are trying not to kill bees that are 50 days old, then because of the t˄2 toxicity time dependence, we need to limit residual concentrations by a factor of almost 2000, or less than 0.4 ppb in nectar and pollen. Honeybees live for more than 150 days when going through the winter, so we have to extend the scaling further and require less than 0.05 ppb to ensure we aren’t killing these wintering bees prematurely. These are exceedingly small quantities of pesticide, well below the level of detection of many assay measurements. These facts demonstrate that it is impossible to effectively limit contamination levels of these chemicals to the low levels that are consistent with protecting long-lived insects while still killing target insects effectively.
Source: Squash Practice, 17 November 2013
http://squashpractice.wordpress.com/2013/11/17/environmental-implicatio…
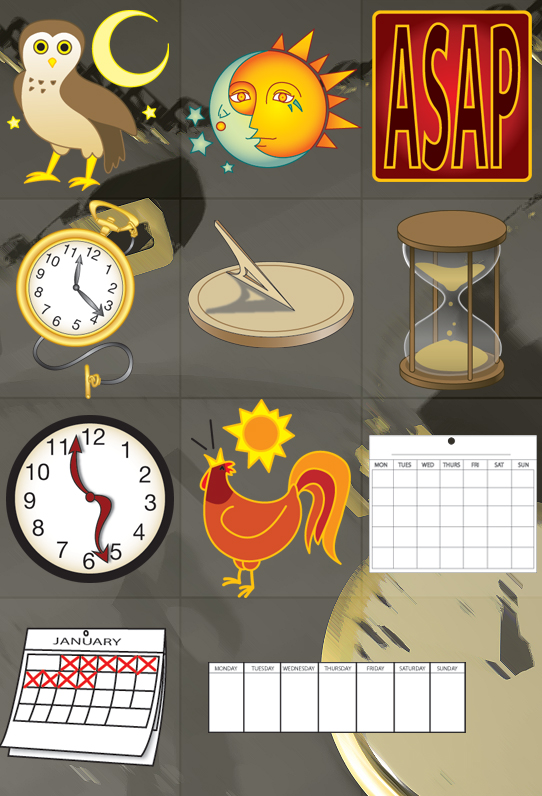
- Login om te reageren